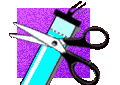 |
Membrane Protein Integrase (MPIase) is an essential component for integrating membrane proteins into the membrane and facilitating the translocation of secretory proteins, which was discovered in the inner membrane of E. coli (1,2). MPIase is a glycolipid composed of 9–11 repeating trisaccharide units consisting of N-acetyl-D-glucosamine, N-acetyl-D-mannosamine uronic acid, and 4-N-acetyl-D-fucosamine, with diacylglycerol linked via pyrophosphate (Fig. 1). There have been no reports of glycolipids being involved in membrane protein transport, and it was important to elucidate the mechanism of action of MPIase at the molecular level. However, obtaining a pure MPIase sample is challenging because it is present in trace amounts in the membrane and heterogeneous in glycan chain length, number and position of acetyl (Ac) groups, and fatty acid types. In addition, the genes and enzymes involved in MPIase biosynthesis are not well understood and MPIase-deficient strains are lethal, making it difficult to elucidate the function of MPIase using molecular biology approaches alone.
Fig. 1 Structure of MPIase
|
Under these circumstances, the chemical synthesis of analogs could be an excellent approach for obtaining structurally defined forms in sufficient quantities. In addition, modified structures can also be obtained. Chemical synthesis of MPIase analogs involves the following challenging points: (i) syntheses of unusual bacterial sugar components, such as fucosamine and mannosamine uronic acid, (ⅱ) construction of β-linked mannosamine, (ⅲ) construction of α-linked glucosamine, (ⅳ) equipment of the Ac group at 6-position of glucosamine, and (ⅴ) introduction of lipids via unstable pyrophosphate linker.
Fig. 2 shows retrosynthetic analysis. These issues were addressed as follows: (i) The fucosamine unit was synthesized from glucose via deoxygenation at C6 and Sɴ2 azidation at C4. (ii) After the construction of the β-linked disaccharide by neighboring group participation of 2-OBz in the glucose donor, Sɴ2 azidation at C2 of the glucose followed by the oxidation at C6 provided mannosamine uronic acid skeleton. (iii) α-linked glucosamine was obtained by S.-C. Hung's approach (3), whose glucosamine donor has an azide group at C2 without a neighboring effect and bulky protecting groups at O3 and O6. (iv) After α-glucosaminylation, the C6 hydroxyl was selectively deprotected by removal of the TBDPS group and acetylated. (v) The monophosphorylated glycan chain reacted with phosphoramidite containing diacylglycerol to form a pyrophosphate linkage.
Fig. 2 Retrosynthetic analysis of MPIase analogs
|
MPIase analogs with various glycan lengths (trisaccharides, hexasaccharides, and nonasaccharides) and modified functional groups (glucosamine 6-OAc, mannosamine carboxy, and pyrophosphate groups) were chemically synthesized using this strategy (Fig. 3a). Structure-activity relationship studies using homogeneous synthetic analogs have revealed the contributions of the 6-OAc group of glucosamine, the carboxy group of mannosamine uronic acid, and the pyrophosphate group to membrane protein integration activity (4,5). Improvements in activity depending on glycan length and the necessity for anchoring of MPIase by diacylglycerol were also confirmed. In addition, a cooperative effect between the MPIase analog and the membrane chaperone YidC was detected. Synthetic analogs were subjected to physicochemical measurements, which revealed the chaperone-like anti-aggregation activity of the glycan moiety for substrate proteins, direct interactions between MPIase and the substrate protein, and an increase in membrane mobility by MPIase analogs (6).
Studies using these synthetic analogs demonstrated a translocon-independent membrane protein integration mechanism in the inner membrane of E. coli as follows: the glycan moiety of MPIase captures a substrate protein synthesized from the ribosome via various functional groups, the secondary structure of the substrate protein is altered to prevent aggregation, pyrophosphate attracts the positive charges of basic amino acid residues in the substrate protein, the hydrophobic interactions between the substrate protein and the membrane cause integration into the membrane, and finally, the substrate protein is handed over to YidC, which enables MPIase to regenerate membrane protein integration activity (Fig. 3b).
Fig. 3 (a) Structure-activity relationship study of MPIase analogs (b) Mechanism of translocon-independent membrane protein integration in E. coli
|
Kohki Fujikawa (SUNTORY Foundation for Life Sciences)
References |
(1) |
Nishiyama K, Maeda M, Nagase R, Yanagisawa K, Komura H, Iwashita T, Yamagaki T, Kusumoto S, Tokuda H, Shimamoto K: MPIase is a glycolipozyme essential for membrane protein integration. Nat. Commun. 3, 1260, 2012 |
(2) |
Fujikawa K, Mori S, Nishiyama K, Shimamoto K: A bacterial glycolipid essential for membrane protein integration. Adv. Carbohydr. Chem. Biochem. 81, 95–129, 2022 |
(3) |
Zulueta MML, Lin SY, Lin YT, Huang CJ, Wang CC, Ku CC, Shi Z, Chyan CL, Irene D, Lim LH, Tsai TI, Hu YP, Arco SD, Wong CH, Hung SC: α-Glycosylation by D-glucosamine-derived donors: synthesis of heparosan and heparin analogues that interact with mycobacterial heparin-binding hemagglutinin. J. Am. Chem. Soc. 134, 8988–8995, 2012 |
(4) |
Fujikawa K, Suzuki S, Nagase R, Ikeda S, Mori S, Nomura K, Nishiyama K, Shimamoto K: Syntheses and activities of the functional structures of a glycolipid essential for membrane protein integration. ACS Chem. Biol. 13, 2719–2727, 2018 |
(5) |
Fujikawa K, Han Y, Osawa T, Mori S, Nomura K, Muramoto M, Nishiyama K, Shimamoto K: Structural requirements of a glycolipid MPIase for membrane protein integration. Chem. Eur. J. 29, e202300437, 2023 |
(6) |
Mori S, Shionyu M, Shimamoto K, Nomura K: Bacterial glycolipid acting on protein transport across membranes. ChemBioChem. 25, e202300808, 2024 |
Jul. 17, 2024
|