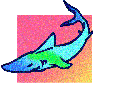 |
Neural plasticity is the ability of the central nervous system to change and adapt in response to an environmental stimulus and experience, and serves as the foundation for memory and learning. Neural plasticity levels in the brain are generally higher in children than in adults. For example, the acquisition of foreign languages is easier for children than for adults. As plasticity decreases with age, it becomes more challenging for an adult to learn a foreign language. This period of high plasticity is called the critical period, and each brain region has its specific timing. Recent research elucidated the molecular mechanisms underlying the onset and termination of the critical period (1).
Chondroitin sulfate (CS), a sulfated glycosaminoglycan, has been shown to act as a molecular brake that terminates the critical period. CS chains are covalently linked to specific serine residues on the core protein and exist as CSPGs. CS chains comprise repeating disaccharide units of glucuronic acid and N-acetylgalactosamine. During biosynthesis, the C4- or C6-hydroxy group of N-acetylgalactosamine residues are sulfated by chondroitin 4-O-sulfotransferase or chondroitin 6-O-sulfotransferase, respectively. 4- and 6-sulfated CS are further modified by other sulfotransferases to form highly sulfated CS. The specific sulfation pattern of a CS chain is considered to encode its biological function (2).
Perineuronal nets (PNNs) are mesh-like extracellular matrices formed around specific neurons. They consist of large aggregates of CS proteoglycans (CSPGs), hyaluronan, tenascins, and link proteins (Figure 1). High- molecular-weight hyaluronan non-covalently binds multiple CSPGs, and link proteins stabilize this interaction (Figure 2). Furthermore, multimerized tenascins associate with CSPGs to form a supramolecular complex. PNNs are selectively formed around parvalbumin-positive (PV) neurons, which play a significant role in neural plasticity in the cerebral cortex. In 2002, Pizzorusso and colleagues demonstrated that an injection of CS-degrading enzyme into the cerebral cortex of adult rats eliminated PNNs and restored neural plasticity (3). Subsequent studies reported that the degradation of CS chains in other brain regions enhanced plasticity. PNNs were recently shown to not only suppress plasticity, but also contribute to the maintenance of memory. Memories acquired through experience and learning in early life are subsequently fixed in neural circuits. The removal of PNNs prevents memory consolidation, which suggests that they are necessary for the long-term protection of memories. In other words, during the critical period when PNNs have not yet formed, new memories are easily acquired due to high plasticity, but once acquired, the formation of PNNs is required for memories to be fixed and maintained in the long term.
Emerging evidence has clarified the molecular mechanisms by which PNNs reduce plasticity. PNNs may function as physical barriers in the perisynaptic space, thus inhibiting synaptic reorganization. PNNs have been shown to suppress synaptic plasticity by inhibiting the lateral diffusion of neurotransmitter receptors in the postsynaptic membrane. Another model indicates that interactions between PNN components and secreted factors affect neural plasticity levels. The secreted homeoprotein Otx2 has been shown to accumulate on the surface of PV neurons by binding to CS chains in PNNs, which is essential for the cellular uptake of Otx2 and the maturation of PV neurons (4). The author and colleagues previously reported that changes in CS sulfation patterns during postnatal brain development were crucial for the formation of PNNs and accumulation of Otx2 (5). Genetically engineered mice with juvenile CS sulfation patterns maintain plasticity as adults. Semaphorin 3A (Sema3A) and Narp, a pentraxin expressed in the brain, regulate neural plasticity by accumulating on the neuronal surface via binding to CS chains. Further studies on the sulfation code of CS chains in neuronal function will provide important insights that will ultimately allow for the future manipulation of neuronal plasticity.
Figure 1. Detection of PNNs around PV cells using Wisteria floribunda agglutinin (WFA)
A PNN visualized by WFA staining (red) showing a lattice-like structure around the soma of a PV cell (green). The merged image shows the vesicular glutamate transporter 2 (VGluT2)-positive synaptic terminals (blue) are embedded in the meshwork of the PNN (red).
|
Figure 2. A schematic model of PNN
CSPGs together with hyaluronan, tenascin-R, and link proteins, form PNNs around synapses. Link proteins stabilize the interactions between CSPGs and hyaluronan. Trimeric tenascin-R cross-links CSPGs and promotes assembly of macromolecular complex in PNNs. CS chains of CSPGs sequestrate several secreted molecules, such as Otx2, Sema3A and Narp, to the surface of PV neurons, which leads to maturation of PV neurons and the termination of the critical period.
|
Shinji Miyata (Graduate School of Agriculture, Tokyo University of Agriculture and Technology)
References |
(1) |
Takesian AE, Hensch TK: Balancing plasticity/stability across brain development. Prog. Brain Res. 207, 3–34, 2013 |
(2) |
Miyata S, Kitagawa H: Formation and remodeling of the brain extracellular matrix in neural plasticity: Roles of chondroitin sulfate and hyaluronan. Biochim. Biophys. Acta Gen. Subj. 1861, 2420–2434, 2017 |
(3) |
Pizzorusso T, Medini P, Berardi N, Chierzi S, Fawcett JW, Maffei L: Reactivation of ocular dominance plasticity in the adult visual cortex. Science 298, 1248–1251, 2002 |
(4) |
Sugiyama S, Di Nardo AA, Aizawa S, Matsuo I, Volovitch M, Prochiantz A, Hensch TK: Experience-dependent transfer of Otx2 homeoprotein into the visual cortex activates postnatal plasticity. Cell 134, 508–520, 2008 |
(5) |
Miyata S, Komatsu Y, Yoshimura Y, Taya C. Kitagawa H: Persistent cortical plasticity by upregulation of chondroitin 6-sulfation. Nat. Neurosci. 15, 414–422, 2012 |
Jun. 15, 2023
|