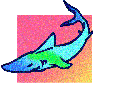 | Heparan sulfate (HS) and heparan sulfate proteoglycans (HSPGs) are expressed by mammalian cells on cell surfaces and deposited in extracellular matrices including the basement membrane. Cell surface HSPGs play a role as receptors for adhesion molecules and growth factors; therefore they are implicated in cell adhesion, migration, differentiation and proliferation. HSPG is a real barrier of the basement membrane and functions as a filter against cationic molecules and macromolecules. HSPGs in the basement membrane also protect a major matrix component, type IV collagen from proteolytic attack. Cell surface HSPGs undergo continuous turnover in which an HS-specific endoglycosidase plays an important role to regulate intracellular metabolism of HSPGs.
In blood borne metastasis, tumor cells disseminate from the primary site, intravasate, circulating in blood vasculature, and then extravasate at a distant organ site. Inflammatory cells also traverse a blood vessel wall and invade into tissues. These metastatic tumor and activated inflammatory cells penetrate the subendothelial basement membrane by enzymatic degradation of large components such as type IV collagen, laminin and HSPGs by a combination of proteases and endoglycosidases. HS-specific endoglucuronidase activity in a normal tissue was first reported by Höök et al. in 1975 (1). HS endoglycosidase activity was also found in fibroblasts, mast cells, and platelets. In 1980Õs a correlation between metastatic potential of tumor cells and their activity to degrade HS in the basement membrane were reported by many laboratories (2). An HS degrading glycosidase found in mammalian cells is an endoglucuronidase, which hydrolyses glucuronosyl glucosaminyl linkages in HS. This endoglycosidase is completely different from bacterial heparitinase, which is an eliminase; therefore we proposed to name the mammalian cell enzyme "heparanase"(3). Heparanase cleaves HS into characteristic large molecular-weight fragments, which need to be analyzed by gel permeation chromatography for detection and qantitation. Due to lack of a rapid assay suitable for a large number of samples for heparanase it took a long time to accomplish its purification. Almost 20 years have passed before the confirmation of a purified human heparanase by cDNA cloning and recombinant enzyme production, and five laboratories almost simultaneously published the purification and cDNA cloning of a human heparanase in the summer of 1999 (4-8).
1. Discovery of heparanase as an extracellular matrix degradation enzyme
In late 1970's Nicolson and his colleagues tried to exploit the molecular mechanisms of organ-specific tumor metastasis and devoted their efforts to investigate tumor-endothelial cell interactions. They finally discovered that highly lung-metastatic mouse melanoma dells underlap vascular endothelial cells and degrade subendothelial basal lamina. The major degradation products released by melanoma cells from subendothelial matrix into culture media were HSPG carbohydrates chains, suggesting that melanoma possess endoglycosidase activity specific for HSPG (9). We further investigated this experimental model and found a good correlation between heparanase activity and lung colonization activity among mouse B16 melanoma sublines (10). The similar results were obtained with various metastatic tumor models such as mouse lymphoma (11) and rat mammary adenocarcinoma (2).
An endoglycosidase found in mouse melanoma was identified as an endoglucuronidase similar to human platelet heparitinase (3). This enzyme was renamed ÒheparanaseÓ to distinguish itself from heparinase and heparitinase, eliminases produced by Flabovaterium heparinum (3). Incidentally, heparitin sulfate is now a dead term in glycosaminoglycan chemistry so that heparitinase is not a preferable name for the newly characterized enzyme specific for HS.
The heparanase is highly active in acidic conditions (pH 4-6.5) suggesting that it is primarily a lysosomal enzyme. However, its activity was detected in cell membranes and in shedded vesicles. Tumor tissues often become hypoxic conditions by insufficient blood supply and undergo acidic conditions around pH 6. Thus, extracellularly released heparanases can easily function on the cell surface or in the extracellular matrix in such locally acidic conditions. An important feature of the mammalian cell heparanase is to recognize very unique domains in HS but not a simple repeat of four saccharides, IdUAGlcN(2-N-sulfate, 6-O-sulfate)-GlcUA-GlcN(2-N-sulfate, 6-O-sulfate) often shown in the biochemistry text books.
2. Purification and cDNA cloning of heparanase
Heparanase activity is not found in stromal and epithelial cells in normal tissues. On contrast blood cells such as platelets and leukocytes possess heparanase activity. It is of interest that virally transformed cells and tumor cells show high levels of heparanase activity in association with malignancy. We used SV40-transformed human embryonic lung fibroblast WI38-VA13 for purification of heparanase (6). Heparanase has strong affinity to its inhibitor, heparin and is glycosylated with high-mannose-type polysaccharide chains. Thus, we used sequential column chromatography with heparin-sepharose and concanavalin A sepharose for purification of human heparanase to homogeneity (6). The purified heparanase showed a single peptide of 50 kDa detected by silver staining after SDS polyacrylamide gel electrophoresis. However, a fragment of 8 kDa derived from C-terminal domain of the proform heparanase also often coexists and could make a hetero dimer with 50-kDa active enzyme (8, 12). We analyzed N-terminal sequences of lysil-endoprotease digests of the purified heparanase, and searched for heparanase-matched cDNA sequences in the EST database. PCR primers for heparanase cDNA cloning were designed based on the sequences found in the EST database, and cloned a cDNA encoding human heparanase from a custom cDNA library of WI38-VA13 cells (6).
The cloned cDNA has an ORF coding for 539 amino acid peptide with 6 potential N-glycosylation sites, which makes 65-kDa protein as a proform. The proform heparanase is converted by unidentified endopeptidases to 8 kDa peptide and 50 kDa catalytic domain with a lysine residue at the N-terminus. The expression of only 50-kDa peptide did not show heparanase activity, suggesting that a propeptide is necessary for formation of the active enzyme (4-8, 12).
The gene of human heparanase is localized in 4q22 and composed of 14 exons and 13 introns (Table 1)(13). In WI38-VA13 cells alternative splicing produces two different size transcripts: 5 kb (HPSE1a) and 1.7 kb (HPSE1b) coding the same peptides (6). High levels of expression of the short transcript are detected in peripheral blood cells and placenta. All laboratories except for us employed a placenta cDNA library resulting in cloning of a short cDNA with 1.7 kb (4, 5, 7, 8). However, heparanases found in platelets and tumor cells were shown to be identical (5).
| |
|
References | (1) | Hook M, Wasteson A, Oldberg A,Biochem Biophys. Res. Commun. 67, 1422-1428, 1975
|
| (2) | Nakajima M, Irimura T, Nicolson GL, J. Cell. Biochem. 36, 157-167, 1988 |
| (3) | Nakajima M, Irimura T, Di Ferrante N, Nicolson GL, J. Biol. Chem. 259, 2283-2290, 1984
|
|
(4) |
Vlodavsky I, Friedman Y, Elkin M, Aingorn H, Atzmon R, Ishai-Michaeli R, Bitan M, Pappo O, Perez T, Michal I, Spector L, Pecker I, Nature Med. 5, 793-802, 1999
|
|
(5) |
Hulett MD, Freeman C, Hamdorf BJ, Baker RT, Harris MJ, Parish CR, Nature Med. 5, 803-809, 1999
|
|
(6) |
Toyoshima M, Nakajima M, J. Biol. Chem. 274, 24153-24160, 1999 |
|
(7) |
Kussie PH, Hulmes JD, Ludwig DL, Patel S, Navarro EC, Seddon AP, Giorgio NA, Bohlen P, Biochem. Biophys. Res. Commun. 261, 183-187, 1999
|
|
(8) |
Fairbanks MB, Mildner AM, Leone JW, Cavey GS, Mathews WR, Drong RF, Slightom JL, Bienkowski MJ, Smith CW, Bannow CA, Heinrikson, RL, J. Biol. Chem. 274, 29587-29590, 1999
|
|
(9) |
Kramer RH, Vogel KG, Nicolson GL, J. Biol. Chem. 257, 2678-2686, 1982 |
|
(10) |
Nakajima M, Irimura T, Di Ferrante D, Di Ferrante N, Nicolson GL, Science 220, 611-613, 1983
|
|
(11) |
Vlodavsky I, Fuks Z, Bar-Ner M, Ariav Y, Schirrmacher V, Cancer Res. 43, 2704-2711, 1983
|
|
(12) |
Parish CR, Freeman C, Hulett MD, Biochim. Biophys. Acta 1471, 99-108, 2001
|
|
(13) |
Dong J, Kukula AK, Toyoshima M, Nakajima M, Gene 253, 171-178, 2000 |
|
(14) |
Hulett MD, Hornby JR, Ohms SJ, Zeug J, Freeman C, Gready JE, Parish CR, Biochemistry 39,15659-15667, 2000
|
|
(15) |
Vlodavsky I, Korner G, Ishai-Michaeli R, Bashkin P, Bar-Shavit R, Fuks Z, Cancer Metastasis Rev. 9, 203-226, 1990
|
|
(16) |
Gohji K, Hirano H, Okamoto M, Kitazawa S, Toyoshima M, Dong J, Katsuoka Y, Nakajima M, Int. J. Cancer in press
|
|
(17) |
Irimura T, Nakajima M, Nicolson GL, Biochemistry 25, 5322-5328, 1986 |
|
(18) |
Nakajima M, DeChavigny A, Johnson CE, Hamada J, Stein CA, Nicolson GL, J. Biol. Chem. 266, 9661-9666, 1991
|
|
(19) |
Saiki I, Murata J, Nakajima M, Tokura S, Azuma I, Cancer Res. 50, 3531-3637, 1990 |
|
(20) |
Mishima T, Murata J, Toyoshima M, Fujii M, Nakajima M, Hayashi T, Kato T, Saiki I, Clin. Exp. Metastasis 16, 541-550, 1998
|
| (21) | McKenzie E, Tyson K, Stamps P, Smith P, Turner P, Barry R, Hircock M, Patel S, Barry E, Stubberfield C, Terret J, Page M, Biochem. Biophys. Res. Commun. 276, 1170-1177, 2000
|
| | |
| |